1.研究背景
1. Introduction
微塑料(MPs)污染在水环境中普遍存在,在一些水生环境中也检测到了非常高的MP浓度(例如,4.5 mg/L)。水生动物意外摄入MPs很常见。许多研究报告称,在不同营养级别的水生生物的消化器官中都含有MPs。然而,目前关于MPs对水生生物的毒理学研究主要集中石油基塑料,如常用的石油基聚合物聚苯乙烯(PS)、聚对苯二甲酸乙二醇酯(PET)和聚氯乙烯(PVC)进行了广泛的研究。而对于近年来不断发展的生物基塑料研究较少。
Microplastics (MPs) pollution is widespread in aquatic environments, and very high MP concentrations (e.g., 4.5 mg·L−1) have also been detected in some aquatic environments.It is very common for aquatic animals to ingest MPs by accident. Many studies have reported that the digestive organs of aquatic biota harvested at various trophic levels contain MPs. The toxicological effects of MPs, such as commonly used petroleum-based polymers of polystyrene (PS), polyethylene terephthalate (PET), and polyvinyl chloride (PVC), on the digestive tract have been extensively studied. However, the effects of the composition of plastic polymers on their toxicological effects remain largely unknown relative to existing information on the effects of their morphology and size.
生物基塑料聚合物被认为是石油基塑料的理想替代品,因为它们可以减少对化石燃料资源的依赖,而且许多此类聚合物可在环境中降解。例如,最常用的生物塑料类型聚乳酸(PLA)已被用于制造食品包装材料、一次性塑料餐具、纺织纤维和生物医学应用的运送载体。PLA的产量多年来稳步增长,预计到2024年将超过30万吨。然而,事实证明,在自然环境条件下,PLA的降解时间比预期的要长。
Bio-based plastic polymers are considered to be ideal alternatives to petroleum-based plastics because they can reduce the reliance on fossil fuel resources and many such polymers are degradable in the environment. For example, polylactic acid (PLA), the most commonly used type of bioplastic, is used to manufacture food packaging materials, has been used to manufacture food packaging materials, disposable plastic tableware, textile fiber, and delivery carriers for biomedical applications. The production of PLA has steadily increased over the years, and is expected to exceed 300 000 t by 2024. Degradable plastics are supposed to completely mineralize in the environment. However, the degradation of PLA has been proven to take longer than expected under natural environmental conditions.
尽管已有研究证实PLA MPs引起成年斑马鱼、幼虫斑马鱼和幼虫蜻蜓行为变化和生化功能障碍,其对水生动物的潜在影响与其可生物降解性有关,并且与传统MPs不同,因此值得进一步研究。PLA MPs可以作为微生物群落的有机碳底物,影响沉积物和土壤微生物群落的组成和功能。摄入的PLA也可能导致宿主肠道中微生物群落的组成和功能发生变化。此外,PLA降解过程中产生的乳酸可能会被动物的肠道菌群同化,从而改变肠道环境的pH平衡,这也会显着影响肠道微生物群落。
Although some behavioral changes and biochemical dysfunctions caused by PLA MPs have been demonstrated in adult zebrafish, larval zebrafish, and larval dragonflies, the potential impacts on aquatic animals related to its biodegradability and different from those of conventional MPs, thereby warranting further study. PLA MPs can serve as organic carbon substrates for microbial communities, and affect the sedimentary and soil microbial community composition and function. Ingested biodegradable PLA might also induce changes in the composition and function of microbial communities in host intestines. In addition, the lactic acid generated during the degradation of PLA might be assimilated by the intestinal microbiota of animals, thereby altering the pH balance of the intestinal environment, which can also significantly impact intestinal microbial communities.
2.研究方案
2. Research Approach
为了确定生物基可降解MPs对水生动物消化道的毒理学影响,将成年斑马鱼暴露于生物基PLA MPs和石油基PET MPs中。比较了鱼类摄入两种不同类型MPs的趋势,并检测了PLAMPs和PET MPs引起的肠道损伤。此外,对鱼肠中的16S rDNA基因进行了高通量测序,以评估PLA诱导肠道菌群失调的潜力,并讨论了与上述生物基可降解MPs的毒性机制。
To identify the toxicological effects of the bio-based degradable MPs on the digestive tract of aquatic animals, adult zebrafish were exposed to bio-based PLA MPs and petroleum-based PET MPs. The tendency of fish to ingest the two different types of MPs was compared, and the intestinal damage induced by PLAMPsand PET MPs was detected. In addition, high-throughput sequencing of the 16S rDNA genes in the fish intestines was conducted to assess the potential of PLA to induce intestinal microbiota dysbiosis. The typical toxicity mechanisms associated with the above bio-based degradable MPs are also discussed.
3.研究结果
3. Results
3.1斑马鱼中MPs的摄入和排泄
3.1. Ingestion and excretion of MPs in zebrafish
在斑马鱼肠道中均检测到PET和PLA。斑马鱼肠道中PET的丰度在第1天为2 ± 4个颗粒,在第5天增加到87 ± 12个颗粒(图 1A)。斑马鱼肠道中检测到的PLA的丰度明显高于PET。这可能斑马鱼可能对PLA的降解过程中产生的乳酸表现出偏好。聚集在混合有5%乳酸的微塑料旁边的斑马鱼数量大约是聚集在不含乳酸的微塑料旁边的数量的两倍。
Both PET and PLA MPs were detected in the zebrafish intestines. The abundance of PET MPs in the zebrafish intestines was 2 ± 4 particles on day 1, and had increased to 87 ± 12 particles on day 5 (Fig. 1 A). The abundance of PLA MPs detected in the zebrafish intestines was significantly higher than that of PET MPs.Zebrafish showed a preference for lactic acid which might be generated during the degradation of PLA MPs. The number of zebrafish that gathered next to the dough mixed with 5% lactic acid was approximately two times greater than the number that gathered next to the lactic acid-free dough.
暴露实验后,将鱼转移到没有MPs的纯水中。鱼肠中的PET和PLA都被迅速排出体外。鱼肠中PET的丰度在1 d内降至13 ± 4个颗粒(图1A)。排泄5天后,仅1~2粒PET MPs留在鱼肠中。同样,鱼肠中PLA的丰度在排泄1 d内显着下降至60±28个颗粒,5 d后99.0%的PLA被排泄出体外。
After the exposure experiments, the fish were transferred to pure water without MPs. Both PET and PLA MPs in the fish intestines were quickly excreted. The abundance of PET in the fish intestines decreased to 13 ± 4 particles within 1 d (Fig. 1A). After 5 d of excretion, only 1–2 particles of PET MPs remained in the fish intestines. Similarly, the abundance of PLA MPs in the fish intestines significantly decreased to 60 ± 28 particles within 1 d of excretion, and 99.0% of PLA was excreted after 5 d.
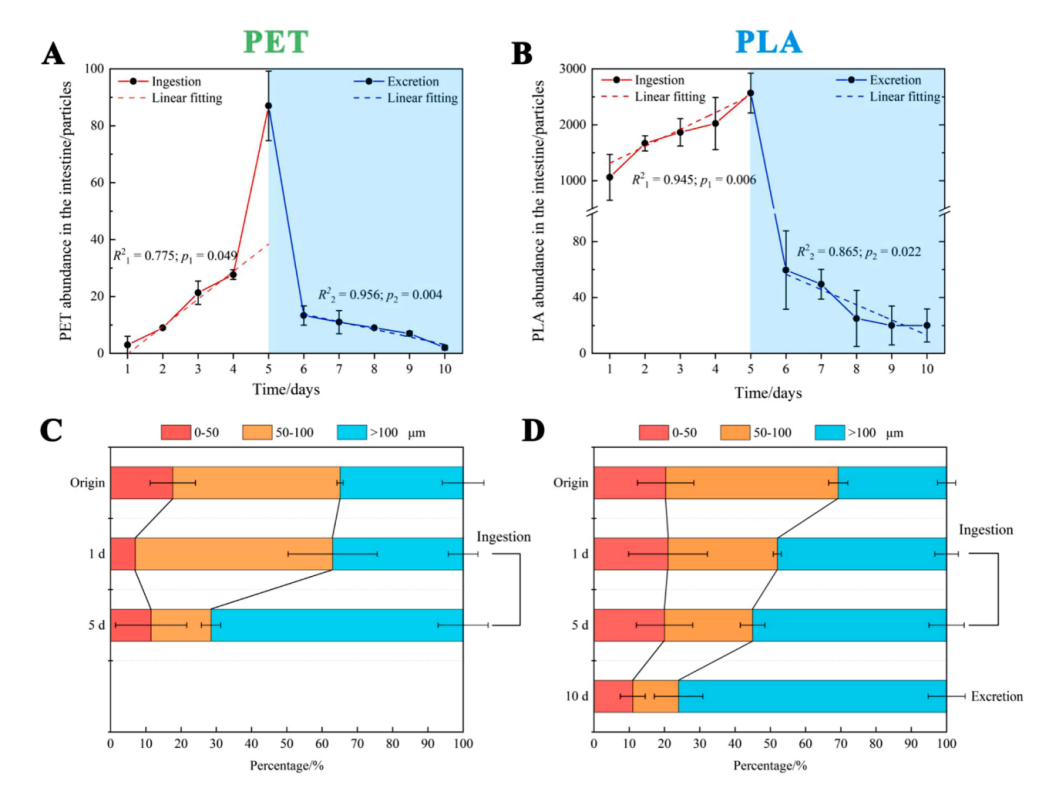
图1斑马鱼肠道中检测到的聚对苯二甲酸乙二醇酯(PET)和聚乳酸(PLA)微塑料(MPs)的数量和大小分布( n = 6)。(A)和(B)中的实线和虚线表示摄入5天(红线)和排泄5天(蓝线)期间鱼肠中MP的丰度。(C)和(D)中不同颜色的列(红色、橙色和亮蓝色)代表原始MP(起源)和鱼肠中MP的尺寸分布(< 50、50~100和> 100 µm)
Fig. 1. Quantity and size distribution of the detected polyethylene terephthalate (PET) and polylactic acid (PLA) microplastics (MPs) in the zebrafish intestines during the ingestion and excretion experiments (n = 6). The solid and dashed lines in (A) and (B) represent the abundances of MPs in the fish intestines during 5 d of ingestion (red lines) and another 5 d of excretion (blue lines). Different colored columns (red, orange, and bright blue) in (C) and (D) represent the size distributions (< 50, 50–100, and > 100 µm) of the original MPs (Origin) and the MPs in fish intestines.
3.2斑马鱼肠道MPs的形态分布和变化
3.2. Morphological distributions and changes in the MPs in zebrafish intestines
本研究使用了具有相似原始尺寸的PET和PLA MPs;大约20%的颗粒小于50 µm,49%为50~100 µm,31%大于100 µm。对于< 50 µm、50-100 µm和> 100 µm的颗粒,第1天在斑马鱼肠道中摄入的PET MPs的尺寸分布分别为7.1%、55.4%和37.5%(图1 C)。暴露5 d后,鱼肠中PET MPs (> 100 µm)的比例增加到69.0%。
PET and PLA MPs with similar original sizes were used in this study; approximately 20% of particles were < 50 µm, 49% were 50–100 µm, and 31% were > 100 µm. The size distribution of the ingested PET MPs in zebrafish intestine on day 1 was 7.1%, 55.4%, and 37.5% for particles < 50 µm, 50–100 µm, and > 100 µm, respectively (Fig. 1C). After 5 d of exposure, the proportion of PET MPs (> 100 µm) in the fish intestines increased to 69.0%.
鱼肠中较大的PLA MPs(>100 µm)的比例也高于水中的浓度,第1天为48.0%,第5天为55.0%(图1D)。在排泄阶段,鱼肠中PLA MPs平均比例< 50 µm和50-100 µm分别下降到10.1%和12.5%,而较大颗粒(> 100 µm)的比例为73.4%。
The proportion of larger PLA MPs (>100 µm) in the fish intestines was also higher than that of the concentrations in water, with values of 48.0% on day 1% and 55.0% on day 5 (Fig. 1D). In the excretion stage, the average proportion of PLA MPs in the fish intestines with sizes of < 50 µm and 50–100 µm decreased to 10.1% and 12.5%, respectively, while the proportion of the larger particles (> 100 µm) was 73.4%.
浸泡在模拟肠道消化液(SIF)中的PET MP的尺寸分布没有显着变化(t值= 0.459,p > 0.05;图2A)。相比之下,PLA MPs的粒径显着减小(t值= 8.087,p < 0.01),尺寸从131. 10 µm(原始)变为92.26 µm(第15天)。
The size distributions of PET MPs soaked in SIF did not significant change (t-value = 0.459, p > 0.05; Fig. 2A). Contrastingly, a significant decrease in the particle size of PLA MPs was observed (t-value = 8.087, p < 0.01), and the median size changed from 131. 10 µm (original) to 92.26 µm (day 15).
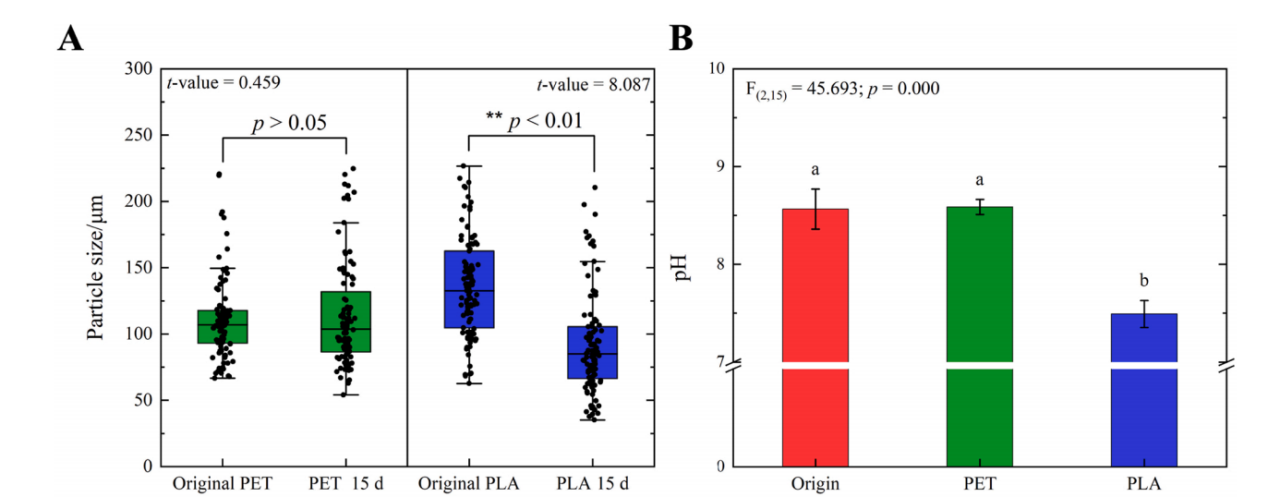
图2模拟肠道消化液(SIF)对微塑料(MPs)的降解作用。A:暴露于SIF 15天后MP大小的变化;B:15天后流体pH值的变化,通过方差分析和Bonferroni事后检验( n = 6 )进行评估。这些值表示为平均值±SD。p值< 0.05被认为具有统计学意义。不同的字母表示实验组之间具有显着差异。
Fig. 2. Degrading action of simulated intestinal digestive fluid (SIF) on microplastics (MPs). A: change in the size of MPs after exposure to SIF for 15 d, assessed by Student’s t-test (n = 100). B: variation in the pH of the fluid after 15 d, assessed by analysis of variance followed by the Bonferroni post hoc test (n = 6). The values are expressed as the mean ± SD. A p-value < 0.05 was regarded as statistically significant. Different letters indicate significant differences between experimental groups.
3.3PET和PLA MPs引起的肠道损伤
3.3. Intestinal damage induced by PET or PLA MPs
在摄入PET和PLA MPs后,在斑马鱼中观察到肠道阻塞。PLA处理中鱼肠的重量显着增加,而PET处理没有增加。在正常的斑马鱼肠道中,绒毛被上皮层覆盖,完整的肠腔内含有对称分布的杯状细胞(图3A)。在暴露于PET MPs的斑马鱼肠绒毛中,空泡化增加(Vac;如黑色箭头所示)和纤毛缺陷(CDs;如红色箭头所示)(图3G);同时,杯状细胞排列无序,丰度下降45.5%(图4B)。在PLA处理中,观察到典型的结构损伤和相对较高的细胞脱落(图3J)。在对照、PET和PLA处理组中,斑马鱼的肠绒毛长度分别为234.9 ± 37.5、256.6 ± 8.3和206.8 ± 3.4 µm(图4 A)。与对照组相比,PLA处理导致肠绒毛缩短19.5%(F (3,20) = 16.454, p = 0.000),鱼肠腔杯状细胞数量减少53.9%。此外,观察到了鱼肠的不良反应包括无序排列的杯状细胞、空泡化、纤毛缺陷和粘液量增加(如绿色箭头所示),这些在PLA处理组中比在PET处理组中更严重(图3J)。
Intestinal blockages were observed in the zebrafish after the ingestion of PET and PLA MPs. The weight of the fish intestines significantly increased in the PLA treatment, whereas it did not increase in the PET treatment. In normal zebrafish intestines, the villi are covered by an epithelial layer containing symmetrically distributed goblet cells in an intact gut lumen (Fig. 3A). In the zebrafish intestinal villi exposed to PET MPs, vacuolization increased (Vac; as indicated by black arrows) and cilia defects (CDs; as indicated by red arrows) were observed (Fig. 3G); meanwhile, the goblet cells were disorderly arranged, and their abundance decreased by 45.5% (Fig. 4B). In the PLA treatment, typical structural damage and relatively high cell shedding were observed (Fig. 3J). The lengths of the intestinal villi in zebrafish in the Ctr, PET, and PLA treatments were 234.9 ± 37.5, 256.6 ± 8.3, and 206.8 ± 3.4 µm, respectively (Fig. 4A). The PLA treatment induced the shortening of intestinal villi by 19.5% (F(3,20) = 16.454, p = 0.000), whereas the number of goblet cells in the intestinal lumen of zebrafish in the PLA treatment decreased by 53.9% compared with that in the Ctr treatment (F(3,20) = 195.996, p = 0.000). The observed adverse effects included disorderly arranged goblet cells, Vac, CDs, and increased mucus volumes (as indicated by green arrows), which were more severe in the PLA treatment than that in the PET treatment (Fig. 3J).

图3连续暴露于微塑料(MPs)15天后成年斑马鱼的肠道形态。A:对照(Ctr)处理中斑马鱼横切肠道切片的整体结构(1:外纵肌;2:内环肌;3:杯状细胞;4:肠绒毛;5:肠腔;6:浆膜;7:淋巴细胞;8:柱状上皮细胞)。B-J:完整的肠、切开的肠和用苏木精和伊红 (H&E)染色的肠切片,(B-D) 为对照组,(E-G)为聚对苯二甲酸乙二醇酯 (PET) 处理组和(H-J)为聚乳酸酸(PLA)处理组。黑色、红色和绿色箭头分别表示肠绒毛中的空泡化(Vac)、纤毛缺陷(CD)和粘液。
Fig. 3. Intestinal morphology of adult zebrafish after 15 d of continuous exposure to microplastics (MPs). A: whole structure of a crosscut intestinal section from zebrafish in the control (Ctr) treatment (1: external longitudinal; 2: internal circular muscle; 3: goblet cell; 4: intestinal villi; 5: intestinal cavity; 6: serosa; 7: lymphocyte; 8: columnar epithelial cell). B–J: complete intestine, cut up intestine, and intestinal section crosscut with haematoxylin and eosin (H&E) staining for the (B–D) Ctr, (E–G) polyethylene terephthalate (PET), and (H–J) polylactic acid (PLA) treatments. Black, red, and green arrows indicate vacuolization (Vac), cilia defects (CDs), and mucus in intestinal villi, respectively.
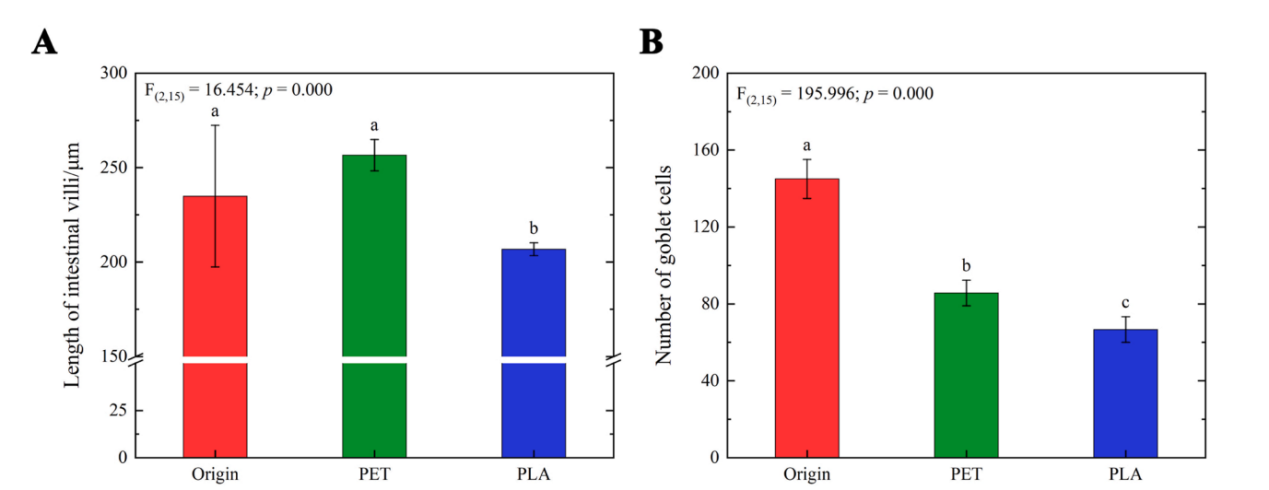
图4微塑料对成年斑马鱼肠道损伤的统计分析(n = 6)。A:每次治疗的横切肠道切片中的肠绒毛长度(µm)。B:每次处理的横切肠切片中杯状细胞的数量。这些值表示为平均值±SD。p值< 0.05被认为具有统计学意义。不同的字母表示实验组之间的显着差异。
Fig. 4. Statistical analysis of intestinal damage induced by microplastics in adult zebrafish (n = 6). A: length of intestinal villi (µm) in the crosscut intestinal section from each treatment. B: number of goblet cells in the crosscut intestinal section from each treatment. The values are expressed as the mean ± SD. A p-value < 0.05 was regarded as statistically significant. Different letters indicate significant differences between experimental groups.
3.5肠道微生物群组成的变化
3.5. Changes in intestinal microbiota compositions
在Ctr、PET和PLA处理中,斑马鱼肠道微生物群中的OTU计数分别为2408、1884和1840。暴露于PET或PLA MPs的鱼肠中的微生物多样性大大减少。PET和PLA处理中独特OTU的计数分别为384和931(图5A)。PCoA结果(图5 B)进一步表明PET样品接近Ctr样品,而PLA样品与Ctr和PET样品有显着区别(R = 0.264,p = 0.046)。因此,与PET MPs相比,PLA MPs对肠道微生物群组成的影响更大。
In the Ctr, PET, and PLA treatments, the OTUs counts in the zebrafish intestinal microbiota were 2408, 1884, and 1840, respectively. The microbial diversity greatly diminished in the fish intestines exposed to PET or PLA MPs. The counts of unique OTUs in the PET and PLA treatments were 384 and 931, respectively (Fig. 5A). The PCoA result (Fig. 5B) further indicated that the PET samples were close to the Ctr ones, whereas the PLA samples were significantly distinguished from the Ctr and PET samples (R = 0.264, p = 0.046). Thus, PLA MPs induced greater changes in the composition of the intestinal microbiota than PET MPs.
肠道微生物群组成的不同可归因于主要肠道细菌丰度的改变。Ctr斑马鱼肠道中的优势门(图5 C)是厚壁菌门(30.3%)、变形菌门(27.4%)、梭杆菌门(22.1%)、拟杆菌门(11.1%)和放线菌门(4.1%)。在PET处理中,这些门在斑马鱼的肠道中也占优势。然而,厚壁菌门和放线菌门的丰度显着降低了15.5% (F (2,9) = 5.374, p = 0.014)和21.0% (F (2,9) = 6.834, p < 0.01)。在属水平上,在PET处理组中,31种肠道微生物群发生了显着变化(图5D)。在PET处理中,鲸蜡杆菌是鱼肠中的优势菌属(21.70%),与Ctr (21.8%)相似。PET中甲基杆菌(F (2,9) = 21.468, p < 0.01)和拟杆菌(F (2,9) = 16.597, p < 0.01)的丰度也大大增加,分别达到13.3%和8.7%,而丰富的乳酸杆菌丰度从3.8% (Ctr)下降到0.5% (PET处理)。这些肠道微生物群主要与鱼类感染和神经退行性疾病有关(图6)。
These distinct profiles of intestinal microbiota composition could be ascribed to the altered abundances of the dominant intestinal bacteria. The dominant phyla (Fig. 5C) in the zebrafish intestines in the Ctr were Firmicutes (30.3%), Proteobacteria (27.4%), Fusobacteria (22.1%), Bacteroidetes (11.1%), and Actinobacteria (4.1%). These phyla were also dominant in the intestines of the zebrafish in the PET treatment. However, the abundances of Firmicutes and Actinobacteria significantly decreased by 15.5% (F(2,9) = 5.374, p = 0.014) and 21.0% (F(2,9) = 6.834, p < 0.01), respectively, in the PET treatment. At the genus level, significant changes were observed in 31 species of the intestinal microbiota during the PET treatment (Fig. 5D). Cetobacterium was the dominant genus in the fish intestines in the PET treatment (21.70%), similar to that in the Ctr (21.8%). The abundances of Methylobacterium (F(2,9) = 21.468, p < 0.01) and Bacteroides (F(2,9) = 16.597, p < 0.01) also greatly increased, to 13.3% and 8.7%, respectively, in the PET treatment, whereas the abundance of Lactobacillus decreased in abundance from 3.8% (Ctr) to 0.5% (PET treatment). These intestinal microbiota that are mainly associated with fish infections and neurodegenerative diseases (Fig. 6).
与PET MPs相比,PLA MPs对肠道菌群的影响更大。PLA处理中的优势门为变形菌门(31.6%)、厚壁菌门(19.2%)、酸杆菌门(12.7%)、软壁菌门(10.4%)和放线菌门(4.1%;图5 C)。此外,酸杆菌门(F (2,9) = 6.672, p = 0.016)和 软壁菌门(F (2,9) = 7.655, p = 0.011)的丰度显着增加,而梭杆菌门的丰度下降了82.0% (F ( 2,9) = 12.564, p = 0.002)。PLA MPs处理的斑马鱼肠道微生物群中的优势属变为支原体(10.4%),而鲸杆菌属、甲基杆菌和乳杆菌分别明显下降至3.9%、1.9%和0.1%(图5 D)。对于与鱼类代谢(包括能量、氨基酸和脂质代谢)、细胞过程(例如细胞生长和死亡、细胞过程和信号传导以及细胞运动)和疾病(感染和神经退行性疾病)相关的微生物群,PLA处理组中斑马鱼的微生物群比PET处理变化更显著(图6)。
PLA MPs induced greater changes in intestinal microbiota than PET MPs. The dominant phyla in the PLA treatment changed to be Proteobacteria (31.6%), Firmicutes (19.2%), Acidobacteria (12.7%), Tenericutes (10.4%), and Actinobacteria (4.1%; Fig. 5C). Additionally, the abundances of Acidobacteria (F(2,9) = 6.672, p = 0.016) and Tenericutes (F(2,9) = 7.655, p = 0.011) significantly increased, whereas that of Fusobacteria decreased by 82.0% (F(2,9) = 12.564, p = 0.002). The dominant genus in the intestinal microbiota of the PLA MP treated zebrafish changed to become Mycoplasma (10.4%), whereas the abundances of Cetobacterium, Methylobacterium, and Lactobacillus clearly decreased to 3.9%, 1.9%, and 0.1%, respectively (Fig. 5D). More significant changes associated with fish metabolism (including energy, amino acid, and lipid metabolism), cellular processes (e.g. cell growth and death, cellular processes and signaling, and cell motility), and diseases (infections and neurodegenerative diseases) were observed in intestinal microbiota of zebrafish in the PLA treatment than in those of zebrafish in the PET treatment (Fig. 6).
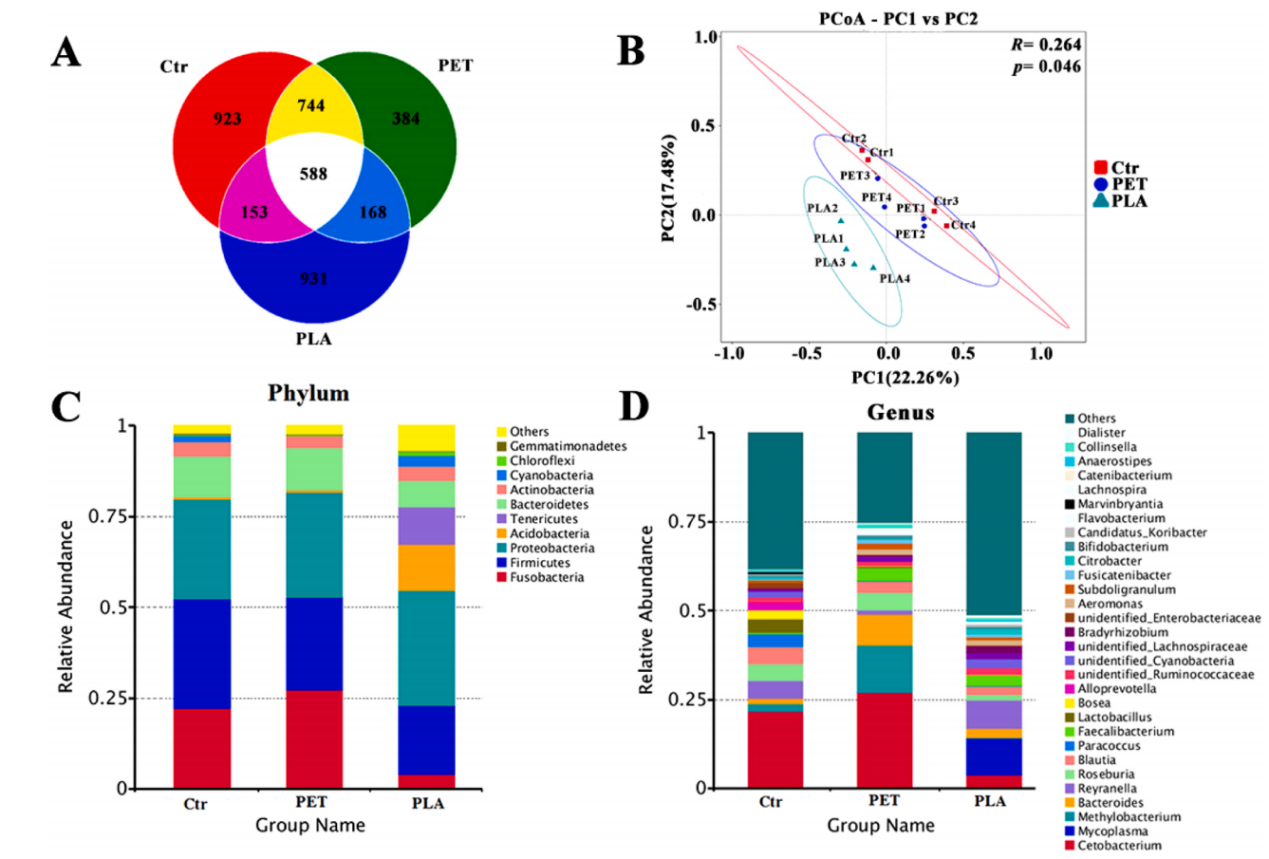
图5微塑料暴露引起的肠道微生物组改变( n = 4 )。A:基于对照(Ctr)、聚对苯二甲酸乙二醇酯(PET)和聚乳酸(PLA)组斑马鱼肠道微生物群中操作分类单位的维恩图。B:肠道微生物组模式的主坐标分析(PCoA)。图中的圆圈代表95%的置信区间。p值< 0.05被认为具有统计学意义。(C)门和(D)属水平的肠道微生物组组成的个体概况。
Fig. 5. Intestinal microbiome alterations induced by microplastic exposure (n = 4). A: venn graph based on operational taxonomic units in the zebrafish intestinal microbiota of the control (Ctr), polyethylene terephthalate (PET), and polylactic acid (PLA) groups. B: principal co-ordinates analysis (PCoA) of the intestinal microbiome patterns. The circles in the plot represent 95% confidence intervals. A p-value < 0.05 was regarded as statistically significant. Individual profiles of the intestinal microbiome composition at the (C) phylum and (D) genus levels.
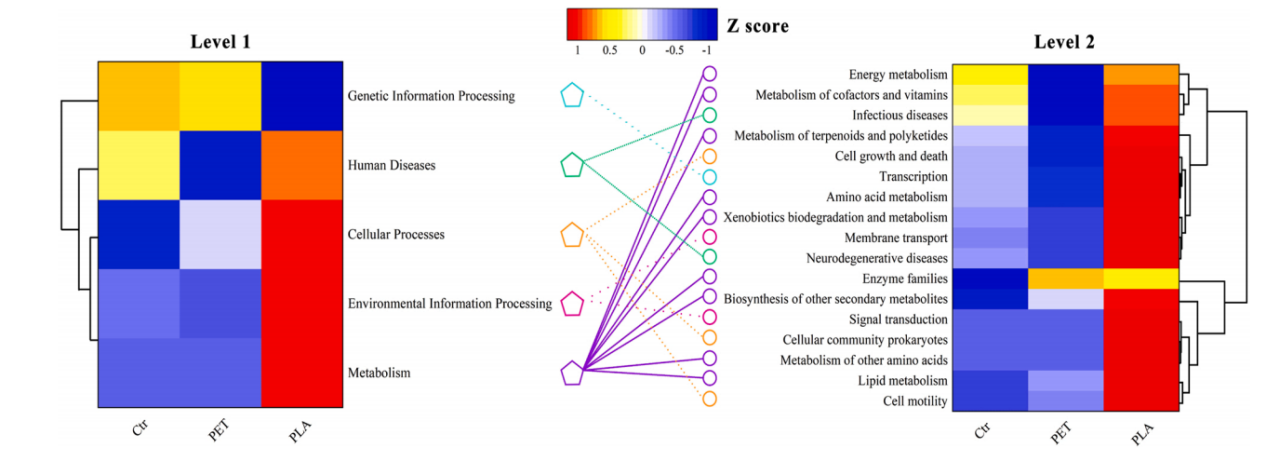
图6连续暴露于聚乳酸(PLA)或聚对苯二甲酸乙二醇酯(PET)15天的成年斑马鱼肠道微生物群的功能注释和聚集。Tax4Fun软件预测了1级和2级功能注释聚类的热图。
Fig. 6. Functional annotation and clustering of intestinal microbiota in adult zebrafish continuously exposed to polylactic acid (PLA) or polyethylene terephthalate (PET) for 15 d. Heatmaps of functional annotation clustering in level 1 and level 2 were predicted by Tax4Fun software.
4.结论
4. Conclusions
与石油基PET MPs相比,生物基PLA MPs更容易被鱼类摄入,对肠道的不良影响更大。PLA MPs暴露还引起肠道微生物群结构和多样性的特定变化,这些变化与鱼类能量代谢和细胞过程的紊乱有关。据推测,在长期暴露下,PLA等生物MP可能通过改变肠道菌群的组成对动物造成更复杂的健康影响,值得进一步关注。
Compared with petroleum-based PET MPs, bio-based PLA MPs were actively ingested by fish, and led to more adverse effects on the intestines. PLA MPs exposure also induced specific changes in the structure and diversity of the intestinal microbiota that were associated with disorders of energy metabolism and cellular processes in fish. It is speculated that under long-term exposure, bio-MPs such as PLA may cause more complex health effects on animals by changing the composition of the intestinal microbiota, thereby deserving further attention.