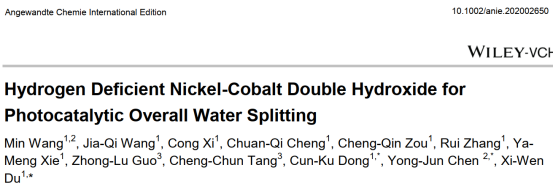
全文速览
Overview
将镍钴双氢氧化物去除部分氢原子,在扩大带隙的同时,暴露出催化析氧反应的钴离子和催化析氢反应的氧位点,在没有牺牲剂和助催化剂的情况下,使单相镍钴氢氧化物实现高效光催化全解水。
By removing part of the hydrogen atoms from the L-NiCo and exposing the cobalt ion forhydrogen evolution reaction (HER) and the oxygen site for the oxygen evolution reaction (OER) while expanding the band gap, the single-phase nickel-cobalt hydroxide is enabled to achieve highly efficient photocatalytic overall water splitting without sacrifice agents and co-catalysts.
背景介绍
Introduction
光解水是一种将太阳能转化为氢能的技术,被视为能源转换和存储领域的“圣杯”。光解水的效率强烈依赖于光催化剂,理想的全解水光催化剂需要满足多种要求:1)要有合适的能带结构吸收太阳光,并能产生具有氧化还原能力的电子和空穴;2)能实现快速的电子空穴分离和传导,3)有良好的析氢和析氧催化活性,4)要廉价稳定高效,便于实际应用。能够同时满足这些的要求的单相催化剂非常少见。迄今为止,全解水的光催化剂通常由两种或者多种材料组成,形成Z型、II型或半导体-金属异质结,通过协同配合实现全解水。然而复合催化剂的工艺复杂、种类有限、价格昂贵,严重限制了大规模应用,迫切需要开发简单高效的单相光解水催化剂。
Photocatalytic water splitting is a promising technique for converting solar energy into renewable H2 energy, in which the photocatalyst plays a key role in improving the conversion efficiency. In principle, an ideal photocatalyst should possess an appropriate band structure to absorb sunlight and to generate electrons and holes with sufficient potentials for redox reactions. In addition, it should facilitate charge separation, present long-term durability, and comprise eco-friendly and earth-abundant materials. Currently, popular photocatalysts consist of two or more components, forming Z-scheme, type II, or semiconductor–metal heterojunctions. Nevertheless, these composite photocatalysts suffer from complicated syntheses, limited material options, and rather high costs, which seriously impedes their large-scale application. Therefore, it is an urgent demand to engineer photocatalysts with simple structures but high efficiencies.
研究的出发点
Starting point of this paper
作为一种常见的电催化剂,过渡金属氢氧化物表现出良好的析氢(HER)和析氧反应(OER)催化能力,低廉的价格也利于大规模的工业应用。但是,过渡金属氢氧化物的带隙过窄,产生的空穴和电子氧化还原能力太差,不足以分解水,拓宽氢氧化物的带隙是一个难题。杜希文团队发现,激光工艺能够创造非平衡的材料,提升催化性能(Nat. Catal., 2019, 2, 1107,Adv. Mater. 2019, 31, 1804769, Adv. Funct. Mater., 2019, 29, 1903444)。在本工作中,我们利用激光合成了非化学计量比的镍钴双氢氧化物(L-NiCo),通过部分去除氢原子,使氢氧化物的带隙展宽,同时暴露出OER催化位点(钴离子)和HER催化位点(氧离子),使单相氢氧化物满足了光催化全解水的要求,获得了优异的全解水性能。
Recently, hydroxides (e.g., NiCo double hydroxides) were found to be promising bifunctional electrocatalysts for the hydrogen evolution reaction (HER) and the oxygen evolution reaction (OER), and their low price facilitates large-scale industrial application. However, their narrow band gaps are insufficient to produce electrons and holes with redox powers that are great enough for water splitting.33-35 Thus far, it has remained a great challenge to broaden the band gap of hydroxide photocatalysts. Du Xiwen’s team found that laser processes can create non-equilibrium materials and enhance catalytic performance. Nonstoichiometric L-NiCo was synthesized by laser ablation in the liquid phase. It displays excellent photocatalytic activity for overall water splitting under sunlight irradiation in the absence of any sacrificial agents and noble-metal co-catalysts. The high performance arises from the nonstoichiometric composition, the adjustable Ni/Co ratio, and the large surface area of this unique photocatalyst. Particularly, nonstoichiometric L-NiCo displays a large band gap and features abundant exposed surface O2− and Co3+ ions. The large band gap enables the photoexcitation of electrons and holes with energies high enough for overall water splitting, while the O2− and Co3+ ions serve as catalytic sites for HER and OER, respectively.
图文解析
材料合成与表征
Synthesis and characterization
利用纳秒激光烧蚀浸泡在碱性(1 M KOH)水溶液中的CoNi合金靶,合成出大量纳米片(命名为L-NiCo,图1a),XRD和元素面分布表明产物是均匀的单相镍钴双氢氧化物(图1b,1c),产物中的Co/Ni的比例可以通过靶材成分来调节。作为对比,我们也用水热方法合成了标准化学配比的镍钴双氢氧化物(命名为H-NiCo)。
The synthetic process is illustrated in Figure 1 a. A nanosecond-pulsed laser was employed to ablate the NiCo alloy target immersed in an aqueous solution of 1 m KOH to fabricate L-NiCo nanosheets (NSs) with a Co/Ni atomic ratio of 83:17. In this process, the metallic target was first heated by the pulsed laser to form a plasma plume of a high temperature (4000–6000 K) and high pressure (1–10 GPa); the metallic plasma then collapsed upon cooling with a liquid medium and reacted with the OH− anions in the solution, leading to numerous hydroxide nanosheets. Meanwhile, the rapid reaction and high cooling rate prevent equilibrium growth, giving rise to a non-stoichiometric product. For comparison, pure Co (OH)2 NSs (L-Co) and pure Ni (OH)2 NSs (L-Ni) were obtained by using pure Co and Ni targets, respectively. Moreover, Ni0.17Co0.83(OH)2 NSs were also prepared by a conventional hydrothermal route, and the product is herein referred to as H-NiCo.
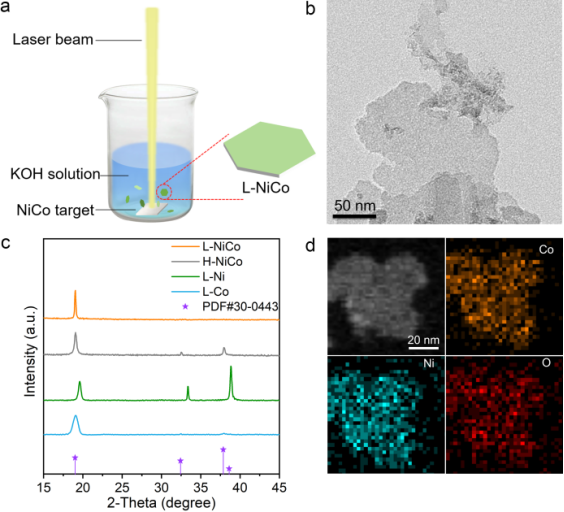
▲图 1. L-NiCo的合成与表征. a) 合成工艺示意图. b)的透射电镜图像. c)主样品和对比样品的XRD 谱图. d) 高角环形暗场透射电镜图像和元素面分布图。
Figure 1 Synthesis and characterization of L-NiCo. a) Synthesis. b) TEM image of L-NiCo. c) XRD patterns of L-NiCo, H-NiCo, L-Co, and L-Ni. d) High-angle annular dark field images of L-NiCo and EDS mapping of Co, Ni, and O.
光解水性能研究
The photocatalytic performance
将 L-NiCo催化剂分散于氢氧化钾水溶液中,在AM 1.5G的模拟太阳光照射下,产氢和产氧的速率分别为1.7 和 0.84 μmol h-1,接近2:1,说明L-CoNi成功驱动了太阳光全解水(图2a)。L-NiCo能在较宽的波长范围吸收太阳光,量子产率随着入射光波长的缩短而增加,从500 nm处的0.37%增加到380 nm处的1.38%(图2b)。Ni/Co金比例也对光解水性能有影响,当Ni含量为17 at%时,H2的产生速率达到最大值。相比而言,水热合成的H-NiCo不显示任何光解水性能。
The photocatalytic performance of the L-NiCo, H-NiCo, L-Co, and L-Ni nanosheets was tested under simulated sunlight irradiation (AM 1.5G) in alkaline aqueous solution without any sacrificial reagents. Upon dispersing 50 mg L-NiCo into 70 mL 1 m KOH solution, the catalyst gave H2 and O2 evolution rates of 1.7 and 0.84 μmol h−1, respectively. The molecular ratio of the produced H2 and O2 was close to 2:1, suggesting successful overall water splitting. After normalization by sample weight, the H2 and O2 evolution rates of the L-NiCo catalysts were calculated as 34 and 16.8 μmol g−1 h−1, respectively; for normalization by surface area, the H2 and O2 evolution rates are 149 and 73.75 μmol cm−2 h−1, respectively. In comparison with previously reported single-phase photocatalysts, our catalyst gave the best photocatalytic performance.
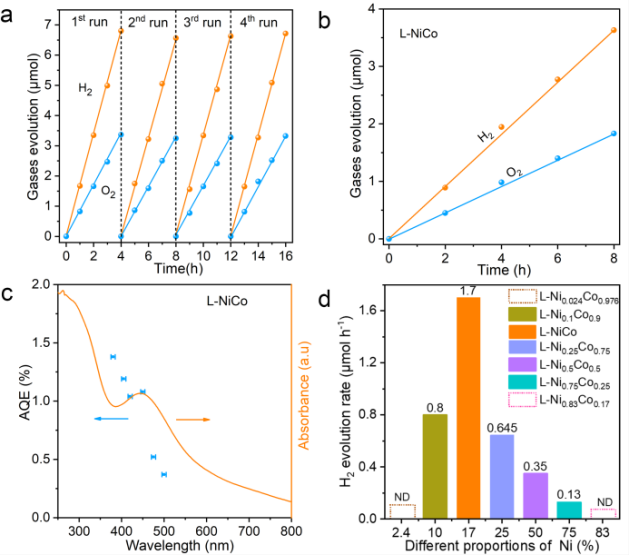
▲图2. L-NiCo的光催化性能。a) AM 1.5G 模拟太阳光下产氢产氧性能。b)波长大于420 nm的可见光下的产氢产氧性能。c) 紫外可见光吸收谱(橘色线) 和外量子效率(蓝色点)。d) 具有不同Ni含量样品的产氢效率,ND: 无法探测。
Figure 2 Photocatalytic performance of L-NiCo. a) Typical time course of H2 and O2 evolution under simulated sunlight (AM 1.5G). b) H2 and O2 evolution under visible-light irradiation with λ>420 nm. c) UV/Vis diffuse reflectance spectrum (solid line) and wavelength-dependent AQE values (symbols). d) H2 evolution rates of double hydroxides with different Ni contents. ND=not detected.
光解水机理探讨
The origin of the photocatalytic performance
吸收光谱表明激光合成的L-NiCo的带隙为3.1 eV 明显大于水热合成的H-NiCo的带隙 (图3a)。Mott-Schottky测试表明L-NiCo和H-CoNi的能级结构均满足光解水的要求,即导带位置在产氢电位之上,价带位置在产氧电位之下(图3c)。荧光光谱和瞬态光电流响应谱说明L-NiCo具有比H-NiCo更强的电荷分离能力,Bode 相图分析表明L-NiCo的电子寿命是H-NiCo的2.36倍。更加重要的是,L-NiCo的HER和OER催化性能均优于H-NiCo,其中,L-NiCO的HER和OER过电势分别为320 和 349 mV@10 mA cm-2,明显低于H-NiCo的 410 和412 mV @10 mA cm-2。正是因为H-CoNi的OER过电势太高,所以光照产生的空穴的势能无法驱动析氧反应进行(图3c),使H-CoNi丧失了光催化全解水的能力。
As shown in Figure 3 a, L-NiCo exhibits an additional absorption peak at around 450 nm overlapped on the absorption baseline, which suggests the possible existence of a mid-gap state arising from the local areas with saturated hydrogen of L-NiCo. The band gap of the photocatalysts can be estimated from Tauc plots.43 As shown in the inset of Figure 3 a, the band gaps of L-Co, L-Ni, H-NiCo, and L-NiCo were determined to be 2.32, 1.94, 2.46, and 3.1 eV, respectively, indicating that laser processing can improve the band gap remarkably. Moreover, the Tauc plot of L-NiCo presents an extra band gap of 2.5 eV between the valence band edge and the mid-gap states, which facilitates the absorption of visible light and is responsible for the hydrogen evolution under visible-light irradiation.We further probe the conduction band edge energy (ECB) by Mott–Schottky measurements (Figure 3 b) and ultraviolet photoelectron spectroscopy. It was found that the ECB of L-NiCo is located at −0.96 V vs. RHE; correspondingly, the valance band edge energy (EVB) could be determined as 2.14 V vs. RHE according to the position of the ECB and the band gap.Moreover, the position of the mid-gap state was estimated as −0.36 V vs. RHE on the basis of the EVB and the mid gap (2.5 V). Notably, the positions of conduction band, mid gap, and valence band meet the requirements for overall water splitting (Figure 3 c). Similarly, H-NiCo possesses an appropriate band structure for overall water spitting. By comparison, the valance band edges of L-Co and L-Ni are higher than the OH−/O2 equilibrium potential, thus the two photocatalysts cannot drive overall water splitting (Figure 3 c).
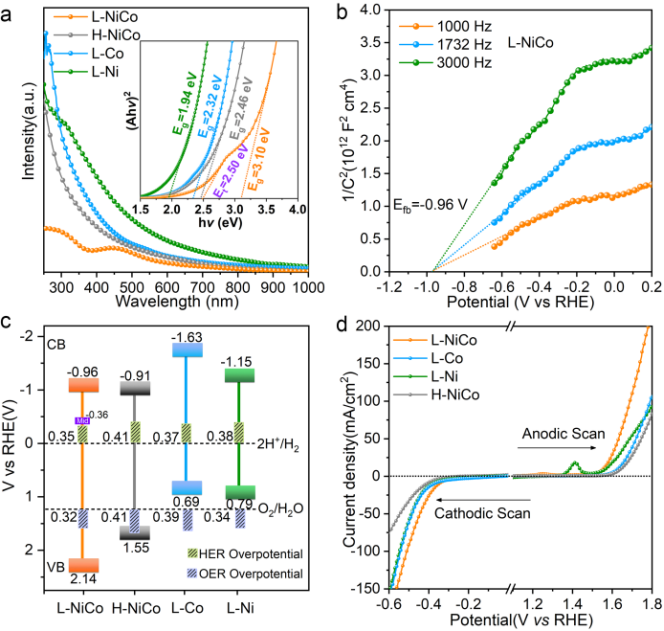
▲图 3不同光催化剂的能级结构。a) 紫外吸收光谱和Tauc图 (插图)。b) Mott-Schottky谱图。c) 能级结构图 (Mid: Mid-gap state)。d)线性扫描伏安曲线(扫速 5 mV s-1 无 iR 校正)。
Figure 3 Energy band structures of different photocatalysts. a) UV/Vis diffuse reflectance spectra and the corresponding Tauc plots (inset). b) Mott–Schottky plots of L-NiCo. c) Energy band diagrams (mid: mid-gap state). d) LSV curves in 1 m KOH solution at a scan rate of 5 mV s−1 without iR correction.
利用X射线荧光光谱(XPS)和X射线吸收近边结构(XANES)对催化机理进行分析,发现L-NiCo中有更多的三价钴(Co3+/Co2+=68%),而H-NiCo中二价钴更多(Co3+/Co2+=25)(图 4a, 4b)。另外,激光合成的L-NiCo中存在大量金属-氧键,而H-NiCo中却没有出现这种化学键(图 4c)。这些结果表明,在激光辐照过程中,L-NiCo中的OH基团被部分破坏,氢原子丢失,导致非化学计量比的NiCo(OH1-x)2。这个结论也得到了拉曼光谱的证明。密度泛函理论(DFT)计算表明,表面氢的部分丢失能够展宽氢氧化物的带隙,并使价带和导带向更高能量移动。
Further, we employed an X-ray photoelectron spectrometer (XPS) to identify the catalytic sites in the photocatalysts. As shown in the Co 2p3/2 spectra (Figure 4 a), the peaks corresponding to Co3+ and Co2+ ions are located at 780.4 eV and 782 eV, respectively. The Co3+/Co2+ ratios of L-NiCo, H-NiCo, and L-Co are remarkably different, namely 47:53 for L-Co, 25:75 for H-NiCo, and 68:32 for L-NiCo. Additionally, X-ray absorption near edge structure (XANES) spectroscopy revealed that the Co K-edge of L-NiCo is located at a higher energy relative to that of H-NiCo, and approaches the Co2O3 reference, suggesting that more Co3+ ions exist in L-NiCo (Figure 4 b). In the Ni 2p3/2 XPS spectra, the Ni2+ and Ni3+ signals are detected at 855.35 and 856.33 eV, respectively, and the Ni3+/Ni2+ ratios for L-NiCo, H-NiCo, and L-Ni are 65:35, 39:61, and 70:30, respectively. Obviously, the concentrations of trivalent metal ions (Co3+ and Ni3+) are higher in L-NiCo and L-Ni. The O1s spectra of the above four samples are shown in Figure 4 c, and the peaks can be deconvoluted into three components related to adsorbed H2O (532.96 eV), lattice OH (M−OH, 531.05 eV), and metal–oxygen bonds (M−CO 529.48 eV), respectively. The peaks from lattice OH and adsorbed H2O can be observed in all four samples, while M−O bonds could only be found in the samples prepared by laser ablation (L-NiCo, L-Co, and L-Ni). These results indicate that lattice OH groups were partially broken and hydrogen atoms were lost during laser irradiation, leading to nonstoichiometric NiCo(OH1−x)2 (x indicates the loss of hydrogen atoms). On the other hand, metal oxide is absent in L-NiCo according to the Raman spectra shown in Figure S25, confirming that the M−O bonds arise not from oxide but from the nonstoichiometric NiCo(OH1−x)2. As described above, nonstoichiometric L-NiCo shows a band gap that is larger than that of stoichiometric H-NiCo, which can be attributed to the loss of H atoms. To confirmed this assumption, we carried out density functional theory (DFT) calculations on a hydroxide model, Co(OH)2. As shown in Figure S26, the partial elimination of surface hydrogen atom leads to an expansion of the band gap from 2.9 to 3.6 eV; meanwhile, the valence band and the conduction band move to higher energies (Figure S27). Therefore, the DFT calculations confirmed the critical role of the nonstoichiometric composition in expanding the band gap.
综合以上结果,单相镍钴氢氧化物的光催化全解水的原因归结为:1)带隙更宽,有足够的产氢产氧驱动力。2)表面暴露的O2-有利于质子吸附和HER过程。(3)Co3+的位点促进了OH-的吸附和OER过程。4)表面吸附的H+和OH-的促进了电荷分离。5)存在中间能隙((mid-gap),有助于光吸收。6)阻抗小,利于电子快速转移。
The nonstoichiometric composition brings about several advantages to the photocatalytic process: 1) Nonstoichiometric L-NiCo possesses a band gap of 3.10 eV, which is larger than that of H-NiCo (2.46 eV), being conducive to the generation of high-energy electrons and holes for overall water splitting. 2) The exposed oxygen atoms on the surface of L-NiCo favor the adsorption of protons from dissociated water molecules and thus boost the HER process. 3) Co3+ sites facilitate OH− adsorption and promote the OER process (Figure 4 d). As a result, HER and OER are triggered at low overpotentials on L-NiCo, making L-NiCo the only photocatalyst that can drive overall water splitting under sunlight irradiation among the four samples studied. 4) The adsorption of H and OH+− on L-NiCo facilitates charge separation, which was confirmed by PL, transient photocurrent response, and electron lifetime analyses. 5) The local areas with saturated hydrogen in L-NiCo bring about a mid-gap state, which contributes to visible-light absorption and hydrogen evolution. 6) Nonstoichiometric L-NiCo exhibits a charge transfer impedance smaller than that of stoichiometric H-NiCo because of the presence of Ni3+ ions, indicating quick electron transfer during HER and OER processes.
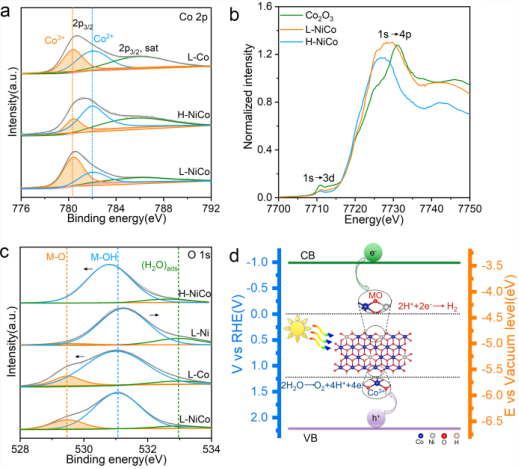
▲图4 L-CoNi催化位点分析。a) Co 2p XPS谱。b) Co K-edge XANES谱。c) O 1s XPS谱。d) 光照下L-NiCo全解水过程示意图
Figure 4 XPS spectra of the as-prepared L-NiCo, L-Ni, H-NiCo, and L-Co samples; a) Co 2p, c) O 1s. b) Co K-edge XANES spectra of L-NiCo, H-NiCo, and Co2O3 standard sample. d) Schematic representation of the overall water splitting process on the L-NiCo photocatalyst.
总结与展望
Conclusion and prospect
激光液相烧蚀合成了非化学计量的L-NiCo,无需任何牺牲剂和助催化剂的帮助,实现了光催化全解水。非化学计量的L-NiCo具有较大的带隙,使电子和空穴的有足够的氧化还原动力,其表面暴露的O2-和Co3+离子,加快了HER和OER反应,从而将惰性双氢氧化物转化为高活性全解水催化剂。我们的工作表明,合理的成分设计可以帮助获得廉价高效的单相全解水光催化剂,同时激光烧蚀是一种强有力的手段,可以用来开发高效的催化剂。
Nonstoichiometric L-NiCo was synthesized by laser ablation in the liquid phase. It displays excellent photocatalytic activity for overall water splitting under sunlight irradiation in the absence of any sacrificial agents and noble-metal co-catalysts. The high performance arises from the nonstoichiometric composition, the adjustable Ni/Co ratio, and the large surface area of this unique photocatalyst. Particularly, nonstoichiometric L-NiCo displays a large band gap and features abundant exposed surface O2− and Co3+ ions. The large band gap enables the photoexcitation of electrons and holes with energies high enough for overall water splitting, while the O2− and Co3+ ions serve as catalytic sites for HER and OER, respectively. Our work suggests that laser ablation is a powerful strategy for converting inactive double hydroxides into highly active catalysts for photocatalytic water splitting, and this technique is promising for developing other simple and cheap photocatalysts.